Numerical models can be used to predict wave overtopping. However, an infinite number of incoming wave time series can be reconstructed from the single wave energy density spectra usually provided. This introduces an epistemic uncertainty which this work will quantify.
This work examines the variability of wave overtopping parameters predicted by numerical models based on non-linear shallow water equations, due to the boundary conditions obtained from wave energy density spectra.
This variability is caused by the free surface elevation time series at the boundary being generated using the principle of linear superposition of spectral components. The component phases are then assumed to be random, making it possible to generate an infinite number of offshore boundary conditions from a single spectrum.
Numerical simulations of reference laboratory tests were carried out. Initial numerical tests consisted of inputting a measured free surface elevation at the toe of a structure during physical modelling, and comparing the resulting individual overtopping volumes. This showed very good agreement between the numerical prediction, the available empirical methods and the overtopping measurements.
A second set of numerical tests was carried out to examine the variability,achieved using measured incident energy density spectra at the toe of the structure, to generate a population of reconstructed offshore boundary time series for each wave condition tested, based on the principle of linear superposition.
An initial seed value is required to generate a population of uniformly distributed random phases. This was obtained using the Monte Carlo technique by varying this value for each simulation. For each wave condition, 500 different time series were generated,allowing a statistical analysis of the results.
Results showed that the variability in the predicted parameters is higher for the smaller number of overtopping waves in the modelled range and decreases significantly as overtopping becomes more frequent. The characteristics of the distributions of the predictions have been studied. The average value of the three parameters has been compared with the measurements. Although the accuracy is lower than that achieved by the model when the measured time series are used at the boundary, the prediction is still fairly accurate, above all for the highest overtopping discharges.
Results indicate that when the probability of overtopping is smaller than 5%, a sensitivity analysis on the seeding of the offshore boundary conditions is recommended.
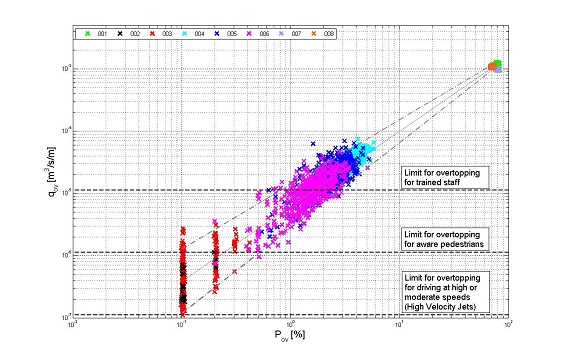
Logarithmic graph showing the correlation between probability of overtopping (Pov,num) and overtopping discharge (qov). Black horizontal dashed lines: overtopping limits from Pullen et al (2007). Solid grey line: trend line of the average qov. Grey dash-dot lines: 95% confidence interval for qov.
Reference: Pullen, T., Allsop, N., Bruce, T., Kortenhaus, A., Schüttrumpf, H., van der Meer, J., 2007. EurOtop - Wave Overtopping of Sea Defences and Related Structures Assessment Manual. Environment Agency, UK.